The rise of nuclear batteries: a revolution in long-lasting power
- Stéphanie
- Mar 7
- 4 min read
Energy sources that are smaller, more efficient, and last longer are on the horizon, and nuclear batteries are expected to become a game-changer. These batteries are miniature power sources, capable of delivering energy for decades without recharging. They could revolutionize multiple industries, from medical implants to space exploration and the Internet of Things (IoT). But what makes these batteries so special, and how do they work?
What are nuclear batteries?
Nuclear batteries, also known as radioisotope batteries or betavoltaic batteries, generate electricity through the decay of radioactive isotopes. Unlike conventional chemical batteries, which rely on electrochemical reactions, nuclear batteries harness energy from alpha (α), beta (β), and gamma (γ) radiation emitted by isotopes such as Plutonium-238, Nickel-63, and Americium-241.
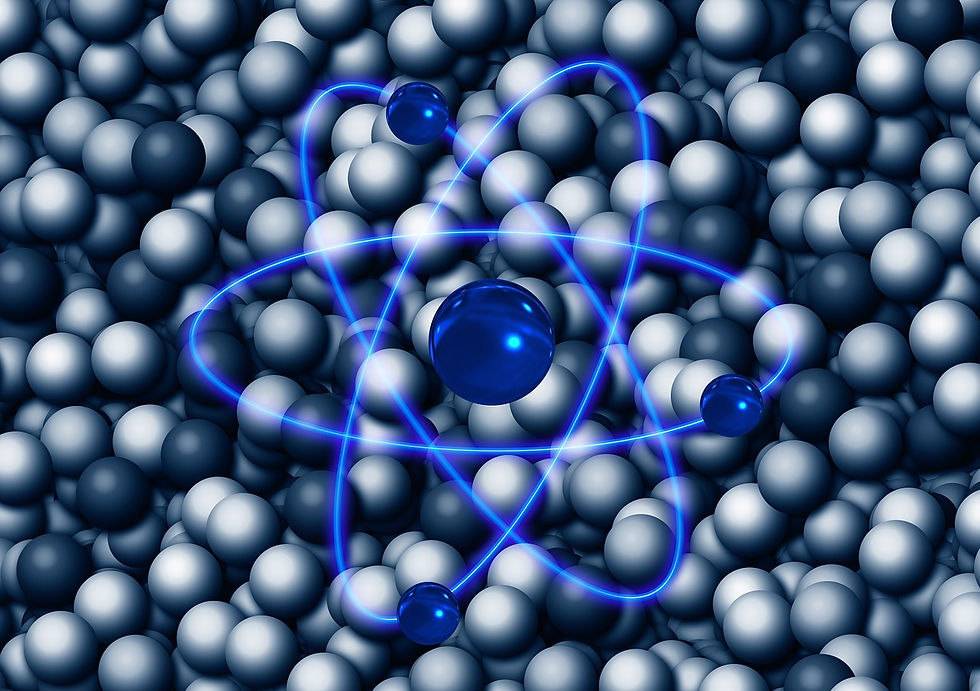
These batteries can last for 50 years or more, making them ideal for applications where battery replacement is impractical, such as space missions, medical devices, underwater sensors, and remote monitoring systems.
How do nuclear batteries work?
At their core, nuclear batteries function using one of the following principles:
Thermoelectric conversion: Radioactive decay produces heat, which is converted into electricity using thermoelectric materials. This is commonly used in space probes such as the Voyager and Mars rovers, which rely on Plutonium-238.
Betavoltaic conversion: Beta particles (electrons) emitted from radioactive decay interact with semiconductor materials (similar to solar cells), generating an electric current. This method is more suited for miniaturized applications like IoT devices and medical implants.
Radiophotovoltaic conversion: A newer approach where radiation excites phosphorescent materials, producing visible light that is then converted into electricity by photovoltaic cells. This technology is currently under development for next-generation MEMS (Micro-Electro-Mechanical Systems).
Recent advances: Rare earth micronuclear batteries
A groundbreaking study published in The Innovation Materials (2024) presents a high-efficiency rare earth micronuclear battery developed by researchers at the Chinese Academy of Sciences. Their innovation involves:
Using a terbium-based luminescent polymer to enhance energy conversion from radioactive decay.
Incorporating Americium-243 as an alpha radiation source, improving efficiency by 8,000 times compared to previous designs.
Designing ultra-compact micronuclear batteries suited for MEMS applications, enabling long-term power solutions for wearable devices, IoT sensors, and space exploration.
This research highlights the potential of rare earth elements in advancing nuclear battery technology, addressing efficiency and miniaturization challenges.
Application of nuclear batteries
Space exploration
NASA and other space agencies have relied on radioisotope thermoelectric generators (RTGs) for decades. Nuclear batteries power rovers, satellites, and deep-space probes where solar energy is insufficient.
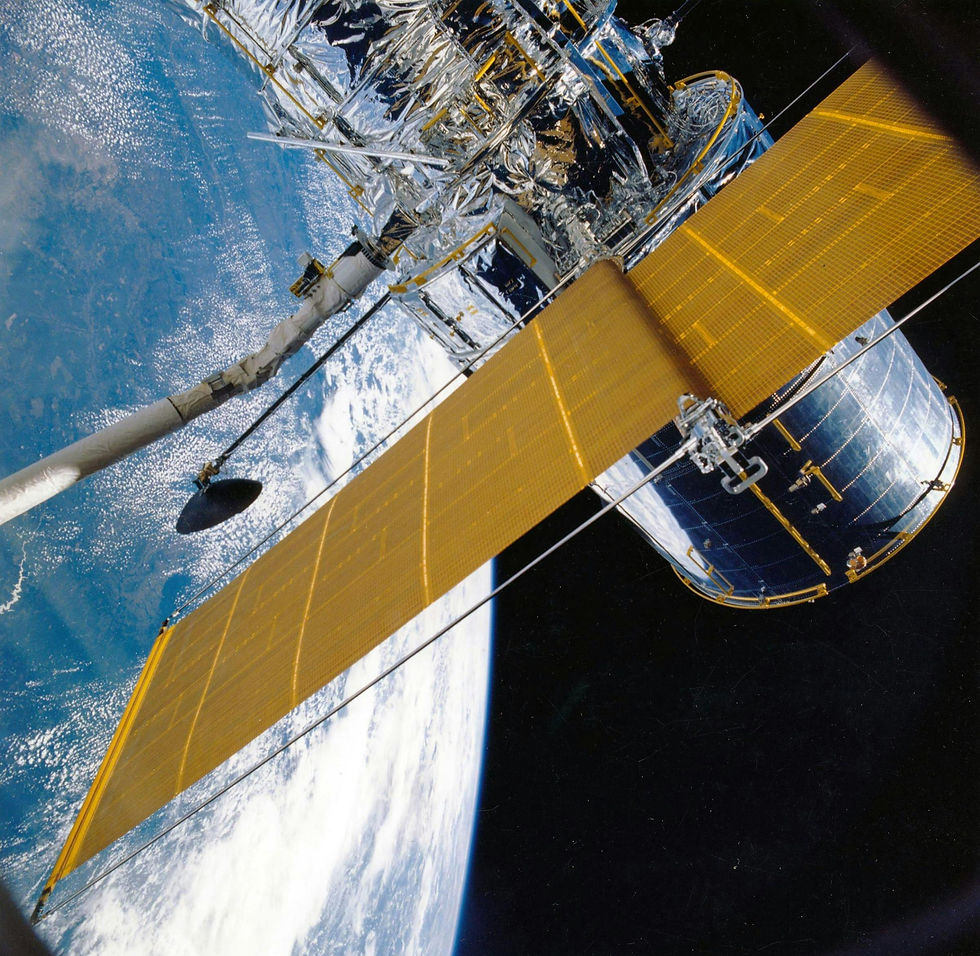
Medical implants
Traditional pacemakers required battery replacements, but nuclear pacemakers, first tested in the 1970s, showed the potential for lifelong energy supply. Modern betavoltaic batteries could power neural implants, artificial hearts, and glucose monitors without needing replacements.
Internet of Things (IoT) and MEMS
The increasing use of autonomous sensors, smart wearables, and industrial IoT demands long-lasting power sources. Nuclear microbatteries could provide energy for decades, eliminating the need for frequent battery replacements in smart cities, defense, and remote monitoring systems.
Underwater and deep-sea applications
For deep-sea exploration and submarine detection systems, where battery replacement is impossible, nuclear batteries offer a stable, long-lasting power source, enabling autonomous underwater vehicles (AUVs) and deep-sea sensors to function indefinitely.
Challenges and controversies
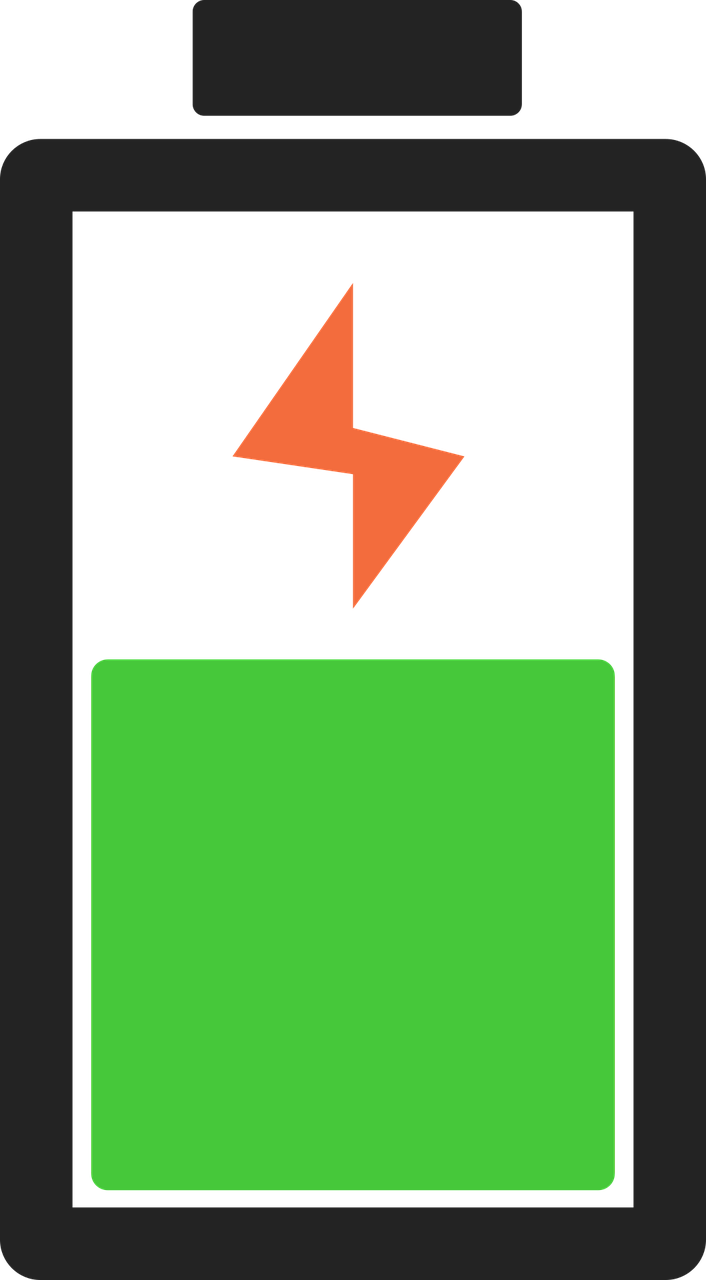
While nuclear batteries offer unparalleled longevity and reliability, they face challenges:
Public perception and safety concerns: The idea of using radioactive materials raises concerns about safety, radiation leaks, and nuclear waste management.
Regulatory hurdles: Strict regulations govern the use of radioactive materials, particularly for consumer applications.
High production costs: Although the efficiency of nuclear batteries is improving, initial manufacturing costs remain high, limiting widespread adoption.
However, advancements in shielding materials, recycling processes, and miniaturization techniques are addressing these concerns, making nuclear batteries more viable for commercial use.
What's next?
With continued research and investment, nuclear batteries could become the ultimate power solution for long-lasting, maintenance-free applications. The latest breakthroughs in rare earth materials, alpha-radioisotope energy conversion, and radiophotovoltaic technologies indicate that we are on the brink of a new energy revolution.
While Iron Man’s arc reactor remains science fiction, nuclear batteries bring us one step closer to a world where energy is virtually limitless, compact, and ultra-efficient. The next decade could see nuclear batteries powering everything from IoT devices to deep-space missions, unlocking a future of uninterrupted power.
Stay updated with NETO Innovation
The world of energy innovation is evolving rapidly, and at NETO Innovation, we are passionate about exploring and sharing the latest breakthroughs in science and technology. To stay informed about cutting-edge advancements like nuclear batteries and beyond:
Subscribe to our blog for the latest insights on innovation and technology trends.
Follow us on LinkedIn to engage with our expert community and industry updates.
References
Rao C., Guo L., Han L., et al. (2024). New high-efficiency rare earth micronuclear battery. The Innovation Materials 2(4): 100104. https://doi.org/10.59717/j.xinn-mater.2024.100104
3. NASA (2023). Radioisotope Power Systems for Space Exploration.
4. Chunlin Zhou et al 2021 ECS J. Solid State Sci. Technol. 10 027005. DOI 10.1149/2162-8777/abe423
Comments